Heat Treatment of Carbon Steel
Heat treatment is a critical process in the manufacturing and processing of carbon steel, a material that is ubiquitous in various industries due to its versatile mechanical properties. The primary goal of heat treatment is to alter the physical and sometimes chemical properties of the material to achieve desired characteristics such as hardness, strength, ductility, and toughness. This article delves into the intricate details of heat treatment processes for carbon steel, exploring the scientific principles, methodologies, and practical applications.
Carbon steel is an alloy of iron and carbon, where the carbon content typically ranges from 0.05% to 2.1% by weight. The presence of carbon significantly influences the mechanical properties of the steel. Lower carbon content results in softer, more ductile steel, while higher carbon content produces harder, stronger, but less ductile steel. Carbon steel is classified into several categories based on carbon content:
- Low Carbon Steel (Mild Steel): Contains up to 0.3% carbon. It is soft and ductile, making it suitable for applications requiring formability and weldability.
- Medium Carbon Steel: Contains 0.3% to 0.6% carbon. It offers a balance between strength and ductility.
- High Carbon Steel: Contains 0.6% to 1.0% carbon. It is harder and stronger but less ductile.
- Very High Carbon Steel: Contains 1.0% to 2.1% carbon. It is extremely hard and brittle, often used for specialized applications like cutting tools.
Fundamentals of Heat Treatment
Heat treatment involves heating and cooling the steel under controlled conditions to achieve specific microstructural changes. The process exploits the phase transformations that occur in the iron-carbon system. The key phases in carbon steel include:
- Ferrite (α-iron): A body-centered cubic (BCC) structure that is soft and ductile.
- Austenite (γ-iron): A face-centered cubic (FCC) structure that exists at higher temperatures and can dissolve more carbon.
- Cementite (Fe3C): An iron carbide that is hard and brittle.
- Pearlite: A lamellar structure consisting of alternating layers of ferrite and cementite.
- Bainite: A microstructure formed by the decomposition of austenite at temperatures below the pearlite range, consisting of ferrite and cementite.
- Martensite: A body-centered tetragonal (BCT) structure formed by the rapid cooling of austenite, resulting in a hard and brittle microstructure.
Heat Treatment Processes
Annealing
Annealing is a heat treatment process that involves heating the steel to a specific temperature, holding it at that temperature for a period, and then cooling it slowly. The primary objective of annealing is to soften the steel, improve its ductility, and relieve internal stresses. There are several types of annealing processes:
- Full Annealing: Involves heating the steel to a temperature above the upper critical temperature (A3) to form austenite, followed by slow cooling to room temperature. This results in a coarse pearlitic microstructure, which is soft and ductile.
- Process Annealing: Used for low carbon steels to improve their cold-working properties. It involves heating the steel to a temperature just below the lower critical temperature (A1) and then cooling it slowly.
- Spheroidize Annealing: Aims to produce a spheroidized microstructure, where cementite particles are spherical rather than lamellar. This is achieved by heating the steel to a temperature just below A1 and holding it for an extended period, followed by slow cooling.
- Stress-Relief Annealing: Used to relieve internal stresses in the steel without significantly altering its microstructure. It involves heating the steel to a temperature below A1 and then cooling it slowly.
Normalizing
Normalizing is similar to full annealing but involves faster cooling rates, typically in still air. The steel is heated to a temperature above A3 to form austenite and then cooled in air. This results in a finer pearlitic microstructure compared to annealing, providing a balance between strength and ductility. Normalizing is often used to refine the grain structure and improve the mechanical properties of the steel.
Hardening
Hardening involves heating the steel to a temperature above A3 to form austenite and then rapidly cooling it, typically by quenching in water, oil, or a polymer solution. The rapid cooling prevents the formation of pearlite and results in the formation of martensite, a hard and brittle microstructure. The hardness of the steel depends on the carbon content and the cooling rate. Higher carbon content and faster cooling rates result in higher hardness.
Tempering
Tempering is a heat treatment process that follows hardening. It involves reheating the hardened steel to a temperature below A1 and holding it for a period, followed by cooling. The objective of tempering is to reduce the brittleness of the martensitic microstructure and improve the toughness of the steel. During tempering, the martensite decomposes into a mixture of ferrite and cementite, resulting in a tempered martensite microstructure. The tempering temperature and time determine the final properties of the steel.
Case Hardening
Case hardening is a surface treatment process that involves adding carbon or nitrogen to the surface of the steel to increase its hardness and wear resistance. There are several case hardening processes:
- Carburizing: Involves heating the steel in a carbon-rich atmosphere to diffuse carbon into the surface, forming a high-carbon case. The steel is then quenched to form a hard martensitic case while the core remains soft and tough.
- Nitriding: Involves heating the steel in a nitrogen-rich atmosphere to diffuse nitrogen into the surface, forming a hard nitride case. Nitriding is typically performed at lower temperatures compared to carburizing, resulting in less distortion and better dimensional stability.
- Carbonitriding: A combination of carburizing and nitriding, involving the diffusion of both carbon and nitrogen into the surface of the steel. This process is typically performed at lower temperatures and results in a hard case with good wear resistance.
Austempering
Austempering is a heat treatment process that involves heating the steel to a temperature above A3 to form austenite, followed by quenching in a salt bath or molten metal bath held at a temperature above the martensite start temperature (Ms) but below the pearlite start temperature (Ps). The steel is held in the bath for a period to allow the austenite to transform into bainite, a microstructure that offers a good balance between strength and toughness. After the bainite transformation is complete, the steel is cooled to room temperature.
Martempering
Martempering, also known as stepped quenching, is a heat treatment process that involves heating the steel to a temperature above A3 to form austenite, followed by quenching in a hot fluid medium held at a temperature just above Ms. The steel is held in the hot medium until the temperature throughout the section is uniform, and then it is cooled to room temperature. This process results in a martensitic microstructure with reduced distortion and improved toughness compared to conventional hardening.
Scientific Principles of Heat Treatment
The scientific principles underlying heat treatment of carbon steel are rooted in the iron-carbon phase diagram, which illustrates the phases present at different temperatures and carbon contents. The phase diagram helps in understanding the phase transformations that occur during heating and cooling, which are critical for controlling the microstructure and properties of the steel.
Phase Transformations
- Austenite Formation: Heating the steel to a temperature above A3 results in the formation of austenite, a face-centered cubic (FCC) structure that can dissolve more carbon. The austenite formation temperature depends on the carbon content of the steel.
- Pearlite Formation: Slow cooling of austenite results in the formation of pearlite, a lamellar structure consisting of alternating layers of ferrite and cementite. The pearlite formation temperature depends on the cooling rate and the carbon content of the steel.
- Bainite Formation: Cooling austenite to a temperature below the pearlite range but above Ms results in the formation of bainite, a microstructure consisting of ferrite and cementite. The bainite formation temperature depends on the cooling rate and the carbon content of the steel.
- Martensite Formation: Rapid cooling of austenite to a temperature below Ms results in the formation of martensite, a body-centered tetragonal (BCT) structure that is hard and brittle. The martensite formation temperature depends on the cooling rate and the carbon content of the steel.
Kinetics of Phase Transformations
The kinetics of phase transformations in carbon steel are governed by the time-temperature-transformation (TTT) diagram, also known as the isothermal transformation (IT) diagram. The TTT diagram illustrates the time required for the transformation of austenite to pearlite, bainite, or martensite at different temperatures. The diagram helps in selecting the appropriate heat treatment parameters to achieve the desired microstructure and properties.
Factors Affecting Heat Treatment
Several factors influence the outcome of heat treatment processes in carbon steel:
- Carbon Content: The carbon content of the steel significantly affects the phase transformations and the resulting microstructure. Higher carbon content results in higher hardness and strength but lower ductility and toughness.
- Alloying Elements: The presence of alloying elements such as chromium, nickel, molybdenum, and manganese can influence the phase transformations and the resulting microstructure. Alloying elements can improve the hardenability, strength, and toughness of the steel.
- Heating and Cooling Rates: The heating and cooling rates during heat treatment significantly affect the phase transformations and the resulting microstructure. Faster cooling rates result in the formation of martensite, while slower cooling rates result in the formation of pearlite or bainite.
- Holding Time: The holding time at the heat treatment temperature affects the phase transformations and the resulting microstructure. Longer holding times result in more complete transformations and more uniform microstructures.
- Quenching Medium: The choice of quenching medium affects the cooling rate and the resulting microstructure. Common quenching media include water, oil, and polymer solutions. The quenching medium should be selected based on the desired cooling rate and the properties of the steel.
Practical Applications of Heat Treatment
Heat treatment of carbon steel is widely used in various industries to achieve specific mechanical properties and improve the performance of components. Some practical applications of heat treatment include:
- Automotive Industry: Heat treatment is used to improve the strength, toughness, and wear resistance of automotive components such as gears, shafts, and bearings. Common heat treatment processes include hardening, tempering, carburizing, and nitriding.
- Aerospace Industry: Heat treatment is used to improve the strength, toughness, and fatigue resistance of aerospace components such as landing gear, engine components, and structural parts. Common heat treatment processes include hardening, tempering, and case hardening.
- Tool and Die Industry: Heat treatment is used to improve the hardness, wear resistance, and toughness of tools and dies. Common heat treatment processes include hardening, tempering, and case hardening.
- Construction Industry: Heat treatment is used to improve the strength, toughness, and corrosion resistance of structural steel components such as beams, columns, and plates. Common heat treatment processes include normalizing, quenching and tempering, and stress-relief annealing.
- Manufacturing Industry: Heat treatment is used to improve the machinability, formability, and weldability of carbon steel components. Common heat treatment processes include annealing, normalizing, and stress-relief annealing.
Advanced Heat Treatment Techniques
Advanced heat treatment techniques have been developed to achieve specific microstructures and properties in carbon steel. Some of these techniques include:
- Austempered Ductile Iron (ADI): ADI is a heat treatment process that involves austempering of ductile iron to achieve a bainitic microstructure. ADI offers a good balance between strength, toughness, and wear resistance, making it suitable for applications such as gears, shafts, and wear-resistant components.
- Induction Hardening: Induction hardening is a surface treatment process that involves heating the surface of the steel using an induction coil, followed by rapid quenching. This process results in a hard martensitic case while the core remains soft and tough. Induction hardening is commonly used for components such as gears, shafts, and bearings.
- Laser Hardening: Laser hardening is a surface treatment process that involves heating the surface of the steel using a laser beam, followed by rapid quenching. This process results in a hard martensitic case while the core remains soft and tough. Laser hardening is commonly used for components such as gears, shafts, and wear-resistant parts.
- Plasma Nitriding: Plasma nitriding is a surface treatment process that involves heating the steel in a plasma environment to diffuse nitrogen into the surface, forming a hard nitride case. Plasma nitriding is commonly used for components such as gears, shafts, and wear-resistant parts.
Quality Control and Inspection
Quality control and inspection are critical aspects of heat treatment processes to ensure that the desired microstructure and properties are achieved. Some common quality control and inspection techniques include:
- Microstructural Analysis: Microstructural analysis involves examining the microstructure of the steel using optical or electron microscopy. This technique helps in identifying the phases present and their distribution, which are critical for understanding the mechanical properties of the steel.
- Hardness Testing: Hardness testing involves measuring the hardness of the steel using techniques such as Rockwell, Brinell, or Vickers hardness testing. Hardness testing helps in assessing the effectiveness of the heat treatment process and the resulting mechanical properties of the steel.
- Tensile Testing: Tensile testing involves measuring the tensile strength, yield strength, and elongation of the steel. Tensile testing helps in assessing the strength and ductility of the steel, which are critical for understanding its performance in service.
- Impact Testing: Impact testing involves measuring the toughness of the steel using techniques such as Charpy or Izod impact testing. Impact testing helps in assessing the resistance of the steel to fracture under impact loading, which is critical for understanding its performance in service.
- Non-Destructive Testing (NDT): NDT involves inspecting the steel for defects such as cracks, inclusions, and porosity using techniques such as ultrasonic testing, radiographic testing, and magnetic particle testing. NDT helps in ensuring the quality and integrity of the steel components.
Environmental and Safety Considerations
Heat treatment of carbon steel involves high temperatures and potentially hazardous materials, requiring careful consideration of environmental and safety aspects. Some key considerations include:
- Emissions Control: Heat treatment processes can generate emissions such as carbon monoxide, nitrogen oxides, and particulate matter. Emissions control measures such as scrubbers, filters, and catalytic converters should be implemented to minimize the environmental impact.
- Waste Management: Heat treatment processes can generate waste materials such as scale, slag, and quenching media. Waste management measures such as recycling, reuse, and proper disposal should be implemented to minimize the environmental impact.
- Safety Measures: Heat treatment processes involve high temperatures and potentially hazardous materials, requiring strict safety measures. Personal protective equipment (PPE) such as gloves, safety glasses, and heat-resistant clothing should be used. Proper ventilation and emergency procedures should be in place to ensure the safety of workers.
- Energy Efficiency: Heat treatment processes can be energy-intensive, requiring efficient use of energy. Energy-efficient furnaces, insulation, and heat recovery systems should be implemented to minimize energy consumption and reduce the environmental impact.
Future Trends in Heat Treatment
The field of heat treatment of carbon steel is continually evolving, driven by advancements in materials science, process technology, and industrial demands. Some future trends in heat treatment include:
- Advanced Materials: The development of advanced materials such as high-strength steels, ultra-high-strength steels, and advanced high-strength steels (AHSS) requires innovative heat treatment processes to achieve the desired microstructure and properties.
- Digitalization: The integration of digital technologies such as sensors, data analytics, and artificial intelligence (AI) can enhance the precision and efficiency of heat treatment processes. Digitalization can enable real-time monitoring, control, and optimization of heat treatment parameters.
- Sustainability: The increasing focus on sustainability and environmental stewardship is driving the development of eco-friendly heat treatment processes. This includes the use of renewable energy sources, energy-efficient furnaces, and waste management practices.
- Additive Manufacturing: The emergence of additive manufacturing (3D printing) technologies is creating new opportunities for heat treatment. Post-processing heat treatment of additively manufactured components can enhance their mechanical properties and performance.
- Nanotechnology: The application of nanotechnology in heat treatment can enable the development of nanostructured materials with enhanced mechanical properties. Nanotechnology can also improve the efficiency and effectiveness of heat treatment processes.
Conclusion
Heat treatment of carbon steel is a critical process that plays a pivotal role in various industries by enhancing the mechanical properties and performance of steel components. The scientific principles underlying heat treatment, including phase transformations, kinetics, and microstructural evolution, provide the foundation for developing and optimizing heat treatment processes. The diverse range of heat treatment techniques, from annealing and normalizing to advanced methods like austempering and laser hardening, offers versatile solutions for achieving specific microstructures and properties.
Quality control and inspection are essential for ensuring the effectiveness of heat treatment processes and the integrity of steel components. Environmental and safety considerations are paramount in minimizing the environmental impact and ensuring the safety of workers. Future trends in heat treatment, driven by advancements in materials science, digitalization, sustainability, additive manufacturing, and nanotechnology, promise to revolutionize the field and open new avenues for innovation.
Reprint Statement: If there are no special instructions, all articles on this site are original. Please indicate the source for reprinting:https://www.cncmachiningptj.com/,thanks!
3, 4 and 5-axis precision CNC machining services for aluminum machining, beryllium, carbon steel, magnesium, titanium machining, Inconel, platinum, superalloy, acetal, polycarbonate, fiberglass, graphite and wood. Capable of machining parts up to 98 in. turning dia. and +/-0.001 in. straightness tolerance. Processes include milling, turning, drilling, boring, threading, tapping, forming, knurling, counterboring, countersinking, reaming and laser cutting. Secondary services such as assembly, centerless grinding, heat treating, plating and welding. Prototype and low to high volume production offered with maximum 50,000 units. Suitable for fluid power, pneumatics, hydraulics and valve applications. Serves the aerospace, aircraft, military, medical and defense industries.PTJ will strategize with you to provide the most cost-effective services to help you reach your target,Welcome to Contact us ( sales@pintejin.com ) directly for your new project.
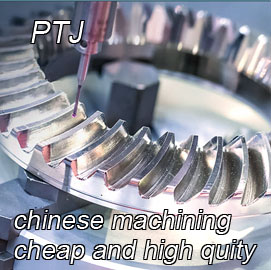
- 5 Axis Machining
- Cnc Milling
- Cnc Turning
- Machining Industries
- Machining Process
- Surface Treatment
- Metal Machining
- Plastic Machining
- Powder Metallurgy Mold
- Die Casting
- Parts Gallery
- Auto Metal Parts
- Machinery Parts
- LED Heatsink
- Building Parts
- Mobile Parts
- Medical Parts
- Electronic Parts
- Tailored Machining
- Bicycle Parts
- Aluminum Machining
- Titanium Machining
- Stainless Steel Machining
- Copper Machining
- Brass Machining
- Super Alloy Machining
- Peek Machining
- UHMW Machining
- Unilate Machining
- PA6 Machining
- PPS Machining
- Teflon Machining
- Inconel Machining
- Tool Steel Machining
- More Material